|
|
|
Introduction
The study of the human gut microbiota and its role in various
diseases has advanced significantly in the last decade. The human
microbiota consists of all microorganisms that live in a symbiosis
with the human body, while the microbiome represents the sum of all
genes of the microbiota.
The human microbiome contains up to 100 times more genes than the
human genome.Microorganisms can be found in various parts of the
human body,but the most numerous is the microbiota of the digestive
organs. The microbiota is variable among healthy people but it is
also unique for an individual – no two microbiomes are the same in
humans, just as there are no the same fingerprints. Although there
are no two people with the same composition of the microbiota,
however, there are larger similarities in the composition of
microbiota among the members of the same races, ethnic groups and
blood relatives.Gut microbiota is a collection of approximately
1014microorganisms. The number of bacteria in every human being is
larger than the number of people who have ever lived on earth.It is
a community ten times more numerous than all the cells of our
organism. It consists of bacteria (about 1000 different species),
archaea, fungi, viruses and parasites that make up a unique
ecosystem. The microbiota plays a very important role in human
health. It is of utmost importance for maintaining the homeostatic
functions of the gastrointestinal tract, as it participates in
digestion processes of the host, metabolism and regulation of the
gut immune system. (1,2). After birth, the digestive tract of the
newborn is not inhabited by microorganisms. In the first hours of
life, it is colonized by maternal microorganisms, initially coliform
bacteria and streptococci, later lactobacilli and enterococci, and
the number of microorganisms in the gut tract begins to increase,
gradually forming a dynamic balance of the gut microbiota.Of course,
the growth of these bacteria also depends on the method of birth -
natural or by caesarean section (3). In adulthood, most of the gut
microbiota consists of five groups of microbes, namely:
Bacteroidetes, Firmicutes, Actinobacteria, Proteobacteria, and
Verrucomicrobia. The number of Gram-positiveFirmicutesand
Gram-negative Bacteroidetesthat make up the most species in a
healthy adult gut> 90% (4-5) is approximately proportional.The ratio
between Firmicutes and Bacteroidetes remains relatively constant in
a healthy individual although it is not the same in all individuals.
The differences occur because of the difference in the host genomes,
environmental factors as hygiene, diet, lifestyle and use of
antibiotics (4). Due to the acidic environment and intense
peristalsis, fewer microorganisms (10–1000 / ml),most of which are
Gram-positive bacteria, are present in the stomach and duodenum.
Enterococci and Lactobacilli are present in the duodenum, and the
number of bacteria in this area is usually 104 / ml. The colon,
which is predominantly inhabited by gram-negative and anaerobic
bacteria, is the richest in the number and variety of species (1012/
ml) (5).
It hasn’t been fully clarified what a healthy microbiota actually
is, but it has been shown that in the case of disturbed balance -
dysbiosis, a disease can develop. When eating habits, environmental
factors, gut infection, some drugs or other factors lead to changes
in the type and amount of gut microorganisms, there occursgut
dysbiosis, which causes inflammatory and metabolic disorders.
Homeostasis of the gut microbiota is crucial for maintaining health
in human beings, while dysbiosis contributes to the development of
various diseases, including cardiovascular, chronic kidney disease,
type 2 diabetes, nonalcoholic fatty liver, and even some types of
cancer (1,6,7). Gut dysbiosis may explain why some individuals are
more prone to developing certain diseases. Changes in the
composition of the microbiota have recently been identified as an
important factor in the dysfunction of the "gut-heart axis", which
contributes to the development of atherosclerosis and hypertension -
two main risk factors for the development of cardiovascular diseases
(1,7,8).
Picture 1. Influence of Intestinal Dysbiosis on
Cardiovascular Diseases(taken from https://www.thelancet.com/journals/ebiom/article/PIIS2352-3964(20)30024-4/fulltext
for scientific purposes and not used for commercial purposes)
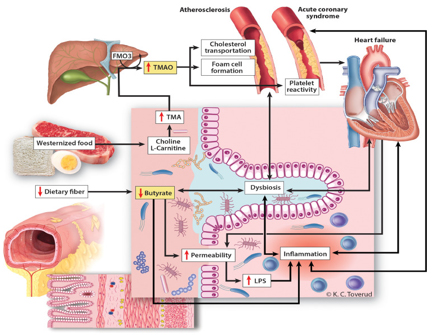
The gut microbiota can be considered an endocrine organ, and each
microbe has the ability to produce hundreds of different known and
unknown metabolites that act beyond the gut itself. As most of the
bacteria that inhabit the digestive organs cannot be colonized in
the laboratory at the moment, for the purpose of determining the
composition of the microbiotanext-generation sequencing method and
bioinformatics analysis of extracted microbial deoxyribonucleic acid
(DNA) are used.In recent years, the impact of the composition of
microbiota on various chronic and autoimmune diseases has been
studied, especially in animal trials (3,8). These trials demonstrate
the importance of the microbiota in relation to health and immunity
and offer new, as yet undiscovered possibilities for the use of this
knowledge in the treatment of some other diseases, such as metabolic
syndrome, insulin resistance, chronic inflammatory bowel disease,
cancer (1,6,9).
Gut dysbiosis andatherosclerosis
Atherosclerosis is the main risk factor for cardiovascular diseases.
This process is characterized by the accumulation of cholesterol and
macrophages (inflammatory cells) in the vascular walls, which
contributes to the formation of atherosclerotic plaques.Recent
studies have shown that gut dysbiosis can contribute to the
development of atherosclerosis by modulating inflammatory processes
and by forming certain microbial metabolites (10-15).Integrity of
the gut mucosa is the first barrier that protects the host from the
intrusion of pathogens, the passage of intestinal contents and
bacterial components into the blood vessels. Reduced concentrations
of proteins that ensure close cell contacts and their impermeability,
including zonula occludens-1 (ZO-1) also known as Tight junction
protein-1 (TJP1), claudin 1, and occludin, allow increased
permeability of the gut wall by disrupting the balance between
mucosal cell death and regeneration of the mucosal cells (1,13,14).
If the mucous barrier is damaged, the penetration of microbes and
their products into the blood vessels triggers an immune response,
tissue and systemic inflammation. Impairment of the gut barrier
integrity caused by gut dysbiosis, therefore, acts as a risk factor
that triggers a chronic inflammation that underlies various
diseases, including atherosclerosis. The main molecules-products of
bacteria that are the drivers of the immune and inflammatory
response are ’’Pathogen-associated molecular pattern’’- PAMP. PAMPs
activate the innate immune response, protecting the host from
infection. A wide range of different types of molecules can serve as
PAMP, including glycans and glycoconjugates. Bacterial
lipopolysaccharides (LPS), endotoxins found on cell membranes of
Gram-negative bacteria, are considered a prototype class of PAMP.The
relationship between plasma LPS levels and cardiovascular risk was
first studied in 1999 by Niebauer et al. (15). The results of the
study confirmed that the level of endotoxemia was the highest in
patients with the most severe cardiovascular disease. Cani et al.
confirmed in their study that gut dysbiosis prevented the formation
of "close contact proteins", which resulted in increased
permeability of gut mucosa, and thus the passage of LPS into the
blood (16). LPS, which are produced in increased amounts in
intestinal dysbiosis, can play an important role in modulating
"toll-like receptors (TLRs)" which recognize bacterial products and
regulate the host's immune system. TLRs are a class of proteins that
play a key role in the innate immune system. They are single-pass
transmembrane receptors commonly found on sentinel cells (first-line
defense cells) such as macrophages and dendritic cells, which
recognize structurally conserved molecules derived from microbes.
Once microbes break through physical barriers such as the skin or
lining of the gut tract, they are recognized by TLRs that activate
immune cell responses.Clinical studies have shown that an increase
in TLR is associated with anti-inflammatory activity and promotes
the development of atherosclerosis in humans. The results of these
studies in recent years thus confirm the role and importance of gut
microbiota and dysbiosis as risk factors in the development of
atherosclerosis (8,9,10,17).
In the metabolism of gut bacteria, various metabolites that
participate in the development of atherosclerosis are formed. Among
the most important are various amines, methylamines, polyamines,
short-chain fatty acids, trimethylamine and secondary bile acids. In
particular, short-chain fatty acids (SCFA) are a group of intestinal
microbial metabolites that are important for metabolic diseases.
Studies have shown that the intestinal microbiota is involved in the
formation of trimethylamine N-oxide (TMAO) (8,14). Trimethylamine (TMA)
is a by-product of bacterial metabolism that is absorbed into the
bloodstream and converted to TMAO in the liver by specific liver
enzymes, flavin-containing monooxygenases. Different bacterial
compositions naturally have different abilities to form TMAO.
Studies in mice have confirmed that TMAO accelerates the development
of atherosclerosis by stimulating cholesterol influx, inhibiting
cholesterol excretion, inhibiting secondary bile acid metabolism,
and / or by excessive platelet activation (3,8,10). According to
researchers, apart from the role of a biological marker for
atherosclerosis and cardiovascular diseases, TMAO could also
represent a possible therapeutic goal in the future. Interestingly,
inhibitors of TMAO production have been developed that target
various microbial TMA lyases. These drugs reduce TMAO levels and
reverse atherosclerosis in animal models. TMA lyase has become the
current potential therapeutic target of TMAO modulation (18).
Gut microbiota and hypertension
Apart from dyslipidemia and atherosclerosis, hypertension is another
major risk factor for CVD that is genetically sensitive and
influenced by environmental factors (19). As early as 1982, it was
shown that antibiotic treatment could cause higher blood pressure
(15). On the other hand, a number of studies showed that antibiotic
use had a beneficial effect on blood pressure.These data, as well as
the observed relationship between dysbiosis and cerebrovascular
events, indirectly suggested a relationship between the gut
microbiota and hypertension as assessed in recent studies.
(20,21).Furthermore, in spontaneously hypertensive rats, a
significant decrease in the number and diversity of microbes in the
gut and a decrease in the number of cecal "good bacteria" from the
species Bacteroidetes was documented, which was accompanied by a
proportional increase in the number of "bad bacteria" from the
species Firmicutes.The studies have also shown that transplantation
of cecal microbiological content from hypertensive animal donors can
reproduce hypertension in previously normotensive animals (22). In a
study on mice, it was shown that dysbiosis of the gut microbiota can
cause angiotensin-II-induced vascular dysfunction and hypertension.
As another study found, the absence of gut microbiota protects mice
from angiotensin II-induced arterial hypertension, vascular
dysfunction, and end-organ damage caused by hypertension (23,24).
Thus, it is obvious that the gut microbiota is involved in the
development or worsening of hypertension. Although the exact
underlying mechanisms and the relationship between the gut
microbiota and hypertension have not been established, existing
evidence from animal trials and clinical studies highlights the role
of short-chain fatty acids -SCFA and oxidized low-density
lipoprotein (ox-LDL) in the development of hypertension. Short-chain
fatty acids, such as acetate, proprionate, and butyrate, are formed
mainly from soluble dietary fiber polysaccharides (23). The groups
of microbes in the gut that metabolize polysaccharides to different
types of SCFA are specific. The main acetate-producing bacteria are
Streptococcus, Prevotella, Bifidobacterium, Clostridiums, and A.
Muciniphila (25). Propionates are produced by Bacteroides,
Salmonella, Dialister, Veillonella, Roseburia, Coprococcus, Blautia,
and others. (26). Butyrates areproduced by Lachnospiraceae,
Ruminococcaci Acid amino coccaceae (27). Fiber and acetate
supplementation led to an increase in the number of Bacteroides
acidifaciens and was associated with improved gut dysbiosis,
hypertension, and heart failure in hypertensive mice (28). Too many
butyrate-producing bacteria have been associated with elevated
systolic and diastolic blood pressure in pregnant women (29).G
protein-coupled receptors (GPCRs) are receptors on the cell surface
that detect SCFA molecules outside the cell and activate cellular
responses. The three GPCRs regulated by SCFA are: GPR41, GPR43, and
GPR109A (30). SCFAs stimulate GPCR-regulated pathways to affect the
renin-angiotensin system to modulate blood pressure. Olfactory
receptor 78 (Olfr78) is another type of GPCR expressed in the kidney
that detects SCFA (31). Both Olfr78 and GPR41 are expressed in
smooth muscle cells ofsmall-diameter blood vessels. In another
study, stimulation of GPR41 resulted in a reduction in the
hypotensive response (32). SCFA, propionate induces vasodilation and
produces an acute hypotensive response in mice by modulating the
activity of Olfr78 and GPR41 (33). All these findings reveal that
the gut microbiota plays an important role in modulating blood
pressure via SCFA and suggests that hypertension is associated with
dysbiosis.
Apart from altered regulation of various receptors via SCFA, gut
dysbiosis also contributes to hypertension by Oxidized LDL-mediated
vasoconstriction(34). Microbial dysbiosis promotes the expression of
pro-inflammatory cytokines and induces oxidative stress that
stimulates LDL oxidation (35). Higher levels of oxidized LDL (Ox-LDL)
reduce NO production, reduce the degree of vasodilation and
stimulate the production of vasoconstrictor substances, including
endothelin-1, which plays a crucial role in maintaining vascular
tone and cardiovascular homeostasis. Disturbed balance leads to
hypertension. However, the causal relationship between gut dysbiosis
and hypertension is complex and has not been fully assessed. The
exact role of the gut microbiota in mediating hypertension, the
pathways and mechanisms involved require further detailed research.
Gut microbiota and heart failure
There is growing evidence of a connection between the gut microbiota
and the pathogenesis of heart failure. In the English literature,
the term "gut hypothesis of heart failure" (36-39) is used to define
this connection. This hypothesis explains that decreased cardiac
output (DCO) and increased systemic arrest can cause gut ischemia
and / or edema of the gut wall, leading to increased bacterial
penetration into blood vessels, thus increasing the concentration of
endotoxins in the circulation. This can trigger inflammation in
patients with heart failure. Exogenous factors such as diet,
exposure to bacterial infections, or medication may reduce the
diversity of the gut flora. Endogenous factors such as acute humoral
imbalance, chronic gut congestion or ischemia-hypoxia, acid-base
imbalance, impaired gastrointestinal motility, and nutritional
deficiency can potentially alter the gut flora (40).With the
development of heart failure, the characteristics of the bacterial
community change. Studies have shown that the number of gut flora in
patients with chronic heart failure decreased, and the number of
pathogenic bacteria increased significantly with the progression of
the disease, including Campilobacter, Shigella, Salmonella, Yersinia
enterocolitica and Candida species (41,42,43). 16SrRNA analysis in
patients with heart failure has reported a reduction in SCFA-producing
bacteria, such as Eubacterium rectale and Dorea longicatena (43).
Another study showed that the composition of the gut microbiota in
chronic heart failure is characterized by a decrease in the number
of bacteria with the potential to produce butyrate (44). Butyrate
exerts local anti-inflammatory effects in the gut mucosa and
stimulates regulatory T cells (45). It has been observed that the
number of microbiological genes for LPS biosynthesis and TMAO
production is increased, while the abundance of genes for butacetate
acetoacetate coenzyme A transferase (key enzyme for butyrate
production) is reduced in chronic heart failure (41).It was also
observed that patients with heart failure and peripheral edema had
higher plasma endotoxin and inflammatory cytokine levels compared
with patients without edema. After short-term diuretic therapy,
serum endotoxin but not cytokine concentrations (46) decreased. In
another study, the researchers confirmed that patients with heart
failure and reduced gut blood flow had higher serum concentrations
of immunoglobulin A - anti-lipopolysaccharide. Compared with the
control group, patients had a different composition of the
microbiota, the number of beneficial bacteria decreased, and the
number of pathogens increased (24). The onset and development of
heart failure may be associated with a decrease in SCFA-producing
bacteria and an increase in TMAO-producing bacteria, which may
become a new target for the treatment of heart failure. Recently, in
studies in mice,the effects of trimethylamine-lyaseenzyme inhibitors
have been shown to have an effect similar to that of anti-atherothrombotic
agents. (47.48).
Gut microbiota and myocardial infarction
Atherosclerotic plaques contain bacterial DNA. However, the types of
bacteria found in atherosclerotic plaques are also present in the
gut of the same individuals (18,19,36). A study from South Korea
shows that the presence of bacteria (microbial rRNA) was detected in
the coronary thrombus during the acute phase of STEMI. The
microbiological signature in the coronary thrombus showed a
correlation with oral and intestinal microbiome (20). From this it
can be concluded that gut microbial communities can be a source of
bacteria in plaque, which can affect plaque stability and the
development of cardiovascular diseases. A recent study in rats
reported a connection between the gut microbiota and the extent of
myocardial infarction (38,39).
The study looked into Dahl Salt-Sensitive Rats-rats fed with foods
high in salt - 8% NaCl) that drank drinking water with antibiotic
vancomycin, which reduced the level ofcirculating leptin by 38%,
causing a smaller myocardial infarction (area reduction of 27%) and
improved restoration of postischemic myocardial contractility
compared to control animals that did not receive the antibiotic.
Vancomycin changed the abundance of gut bacteria and fungi measured
by the amount of 16S and 18S rRNA (39).
In rodent-based studies the use of Lactobacillus plantarumas a
probiotic resulted in the reduction of circulating leptinby 41%,
myocardial infraction by 29% and better recovery of myocardial
contractile function by 23%.However, if rodents received leptin at a
dose of 0.12 µg / kg i.v. the protective effect of probiotics on the
heart was reversed. This study is the first to confirm a direct link
between changes in the gut microbiota and myocardial infarction.
This shows that the addition of probiotics can reduce the degree of
myocardial infarction (46). Another animal study using Lactobacillus
rhamnosus showed a beneficial effect on cardiac function after an
artificially induced myocardial infarction (49).
Gut microbiota and metabolic diseases
Many studies show a link between the composition of the gut
microbiota and metabolic disorders in the body (50,51,52). The role
of the gut microbiota in the development of obesity has been proven
by studies conducted on dehydrated mice (germfree-GF-mice) compared
with conventionally bred mice (CONV-R). Sterile, germ-free mice are
bred in isolators that completely block exposure to microorganisms,
with the intention of protecting them from detected bacteria,
viruses, and eukaryotic microbes.CONV-R mice have a 40% higher body
fat content than GF-mice, which is a phenomenon independent of food
intake. However, after colonization of GF-mice by gut flora coming
from CONV-R mice, a significant increase in body weight and ~ 60%
increase in body fat was observed, together with increased synthesis
of liver triglycerides in faecal transplant recipients (GF mice),
independent of food intake and total energy consumption (53). It
appears to be the mechanism by which gut microbes contribute to
increased energy absorption by the formation of short-chain fatty
acids (SCFA), which is the result of hydrolysis and fermentation of
dietary polysaccharides. SCFAs, such as propionate, butyrate, and
acetate, perform complex metabolic actions that affect host
appetite, gut transittime, and fat absorption and deposition
(52).SCFAs also increase the internal absorption of monosaccharides
by stimulating the expression of the sodium-glucose transporter 1.
SCFAs also contribute to the modulation of host appetite and food
intake in interaction with G-linked proteins expressed by
enteroendocrine cells and promote the release of glucagon-like
peptide-1 that affects satiety. Apart from that, SCFAs affect lipid
metabolism by increasing lipogenesis and inhibiting fatty acid
oxidation (53).Studies have shown specific changes in the
composition of the gut microbiota in genetically obese mice compared
to genetically lean mice, showing a 50% decrease in abundance of
Bacteroidetes and a proportional increase in Firmicutes. These
specific changes appear to contribute to increased SCFA production
and fat accumulation in obese mice and in GF mice colonized by obese
microbiota (54). There are other possible mechanisms. A high-fat
diet has been shown to increase the proportion of Gram-negative
species in the gut microbiota, which contributes to the increased
absorption of gut fragments of bacteria, such as lipopolysaccharides
(LPS) in the gut. Increased circulating LPS levels lead to
"metabolic endotoxemia" which manifests as weight gain, fasting
hyperglycemia, and hyperinsulinemia (55). There is growing evidence
to suggest that a high-fat diet promotes changes in the composition
of the gut microbiota, but the later development of the obesity
phenotype is associated with metabolic endotoxemia (56).In recent
years, researchers have also studied the links between dysbiosis and
obesity, type 2 diabetes, dyslipidemia, and nonalcoholic fatty liver
disease (NASH) (50,51). Initial studies in animals and humans
supported a correlation between obesity and the abundance of the
Firmicutes group of bacteria compared to the Bacteroidetes group;
type 2 diabetes, however, is associated with a reduced abundance of
butyrate-forming bacteria and an increased abundance of
Lactobacillus spp (1,3,8,10). The gut microbiota is involved in the
development of dyslipidemias via secondary bile acids (52,53). In
the research of NASH, it was determined that some bacteria
(Clostridium coccoides, Lactobacillus reuteri, Parabacteroides)
affect fat metabolism, integrity of the gut wall and the process of
fibrosis, thus affecting dyslipidemia (52).
Application in practice
Examples of clinical benefit of long-term microbiota change are:
dietary measures, pre and probiotic therapy, antibiotic therapy,
intake of targeted enzyme inhibitors, fecal microbial
transplantation, etc. (57,58). Studies have shown that even a
five-day change in diet leads to a short-term rearrangement of the
number and types of gut microbes (4). An example of this is the
dietary approach aimed at stopping hypertension (DASH diet - Dietary
Approaches to Stop Hypertension), which consists of meals with
fruits, vegetables, whole grains, etc. (59).Patients in the study
who were on this diet showed an improvement in quality of life and
better elasticity of arterial blood vessels after three months of
adherence to the measures (60). Besides, it has been described that
individuals who do not follow a prescribed diet and have a “Western
diet” high in fat and red meat have elevated levels of TMAO in their
urine compared to patients who follow a prescribed DASH regimen
(61,62).Reduced dietary fiber intake is associated with reduced
bacterial production of short-chain fatty acid butyrate, which has
immuno-modulatory effects in the gut mucosa and also serves as a
major energy substrate for colonocytes.Decreased levels of butyrate
in the gut could induce local inflammation, worsen dysbiosis and
contribute to impaired gut barrier function, resulting in "leakage"
of bacterial toxins such as LPS, which further induces local and
systemic inflammation. A high-fiber diet can improve the growth of
acetate-producing bacteria, reduce high blood pressure, and prevent
heart fibrosis and hypertrophy (63).
Probiotics and prebiotics
Probiotics are living microorganisms which, when given in
appropriate amounts, bring health benefits to the host (64).
Probiotics in clinical use contain bacterial and fungal
microorganisms, including the genera Lactobacillus and
Bifidobacterium and the fungus Saccharomyces boulardii (65). The
results of animal models suggest that certain strains of
lactobacilli could have cardioprotective effects. Rats treated with
a supplement containing Lactobacillus plantarum 299v prior to
coronary artery ligation reduced infarct size and improved left
ventricular function (66).Another study showed similar
cardioprotective results in a rat model of myocardial ischemia after
supplementation with Lactobacillus rhamnosus GR-1 (67). A pilot
study in humans reported not only reduced systemic inflammation, but
also improved ejection fraction after intervention with probiotic
yeast Saccharomyces Boulardii in patients with chronic heart failure
(68). Given the potential clinical impact of microbiota modulation,
as well as high morbidity and mortality from heart failure,
microbiota modulation is not completely risk-free (69). Careful
clinical monitoring and pre-defined safety measures which should
follow the same standards as in other clinical trials are
recommended, (70) because recent genomic and epidemiological
evidence of probiotic-related bacteremia or transfer of bacteria
from probiotic capsules into the blood of patients in intensive care
units has been reported (71).Prebiotics are substrates that
microorganisms of the host selectively use and provide potential
health benefits. Dietary fiber and oligosaccharides are most
commonly used as prebiotics (72). Most modern studies that deal with
microbiota processing in patients with cardiovascular disease report
NAPOMENA – NEJASNO microbial depletion with SCFA-forming capacity
such as butyrate. Prebiotics that promote microbiological
fermentation of dietary fiber in SCFA may, therefore, be of
potential benefit in improving metabolic regulation (73). Some
prebiotics, such as inulin, have the potential to counteract the
harmful effects of antibiotics by promoting the diversity and
functional capacity of the gut microbiota (74).A randomized study
with inulin food supplement or inulin-proprionate ester showed a
decrease in markers of systemic inflammation with increased
generation of SCFA proprionate in the colon (75). Therefore,
targeting the production of microbial SCFAs with inulin supplements
or other prebiotics is an attractive strategy for future
cardiovascular disease testing, although current scientific evidence
does not provide validated recommendations for the use of probiotics
or prebiotics as adjunctive therapy in patients with heart failure
or coronary heart disease.
Antibiotics
The use of antibiotics affects the composition, diversity and
function of the normal flora (76). Antibiotics have been used
successfully in animal models to reduce the degree of damage to
heart cells after myocardial infarction (77,78). Previous studies in
patients with heart failure focused on gut decontamination with
broad-spectrum antibiotics to reduce biotome translocation and
bacterial inflammation. Although this approach succeeded in reducing
markers of systemic inflammation, a clinical effect was not
demonstrated (79,80).
A recent study showed that a broad-spectrum oral antibiotic cocktail
significantly increased postinfarction rupture and death in a mouse
model of coronary artery ligation (81), which could suggest that an
intact microbial community is needed for proper myocardial recovery
at the time of myocardial injury. This study is in contrast to a
previous animal experimental model that showed that oral vancomycin
reduced infarct size and improved postinfarction cardiac function in
rats (82), and to a study reporting that a combination of
streptomycin, neomycin, polymyxin B, and bacitracin reduced infarct
size along with changes in metabolites associated with the
microbiota (83).
Regardless of the differences, these animal studies strongly
indicate the role of gut microbiota composition in acute myocardial
infarction, but the direction of microbiota changes and potential
metabolic or inflammatory pathways are not yet well known. Modifying
cardiovascular diseases with antibiotic therapy is not a new idea.
Between 1995 and 2005,> 19,000 patients were included in a study to
treat pneumonia in patients with coronary heart disease. The study
in these patients showed no clinical benefit from antibiotic therapy
in relation to coronary ischemia (84). Apart from the apparent risk
of antimicrobial resistance, other safety concerns have recently
emerged with potential significance for future testing. A recent
ten-year follow-up showed increased cardiovascular death in patients
with stable coronary heart disease treated with clarithromycin (85),
leading to a 2018 FDA warning on the use of clarithromycin in
patients with coronary heart disease. The FDA advises caution before
prescribing clarithromycin antibiotic to patients with heart disease
because of a potential increased risk of heart problems or death
that may occur years later.(https://www.fda.gov/drugs/drug-safety-and-availability/fda-drug-safety-communication-fda-review-finds-additional-data-supports-potential-increased-long).
In December 2018, the FDA issued a warning on the use of
fluoroquinolones indicating the possibility of aortic rupture and
aortic dissection in high-risk patients, such as elderly patients
with hypertension or peripheral atherosclerotic vascular
disease(https://www.fda.gov/drugs/drug-safety-and-availability/fda-drug-safety-communication-fda-review-finds-additional-data-supports-potential-increased-long).Another
study reported increased risk of cardiovascular events in older
women with increased cumulative antibiotic exposure in adulthood
(86). The explanation for this increased risk is not fully known in
all patients, but it could include QT prolongation, arrhythmias or
pro-inflammatory activities mediated by gut microbiota
translocation, or other effects mediated by the gut microbiota.
Considering these safety concerns and the lack of a completely clear
clinical effect antibiotics have on the microbiota, caution should
be exercised in future studies targeting the use of antibiotics in
cardiovascular patients.
Targeted enzyme inhibitors
Apart from the above-mentioned use of TMA lyase (18), mention should
be made of the results of a study in which mice used choline analogs
that inhibit the action of enzymes in TMA metabolism, thereby
reducing plasma TMAO concentrations. The use of choline analogues
could, therefore, provide a new approach to reducing the risk of
thrombosis (69). Another interesting active ingredient that acts as
a protective factor for the gut mucosa is Urolitin A (UroA) and its
synthetic analogue UAS03, which improve close cell contact and
gastrointestinal barrier function (87).
In recent years, fecal microbial transplantation (FMT) has been
among the most mentioned interventions used to treat intestinal
dysbiosis. The introduction of "good bacteria" taken from healthy
subjects into the gastrointestinal tract of patients suffering from
gut dysbiosis and its consequences is a new and effective
therapeutic strategy. In a clinical study examining people with
metabolic syndrome, there was a significantly improved insulin
sensitivity after 6 weeks of FMT in which the donors were healthy
people of normal weight. At the same time, FMT increased the amount
of butyrate-producingbacteria (88). Although the acceptance of the
therapeutic use of FMT is increasingly present, due to the
perception of this method as a "natural" treatment and relatively
cheap application, the risk-benefit ratio particularly in CVS
diseases remains insufficiently clearly defined because the
published experience with FMT is limited and the legal norm of this
therapy has not yet been precisely regulated. Furthermore, there is
a fear of the infectious potential of the therapy, which led
researchers to investigate the use of "synthetic stool" products
with a defined population of bacteria to alleviate such problems,
and the use of "frozen donor material" such as the concept of a stem
cell bank is being considered (89).
Concluding remarks
New knowledge and technologies are significantly changing medical
doctrine, enabling a new, different view of the body, organs and
health, as well as the causal factors of diseases. Research in the
recent past, and sometimes surprising findings, have confirmed that
the gut microbiota can affect the health of the host and trigger the
disease by means of various pathophysiological mechanisms. Gut
microbiota and dysbiosis are areas of research which,in the
future,willlikely change the established methods of prevention and
treatmentof diseases.
Although we can change the composition of the microbiota with
prebiotics, probiotics, antibiotics, diet and "targeted enzyme
inhibitors", for the time being we cannot predict and provide a
detailed assessment of all the effectsof these interventions in the
prevention of various diseases. With all the data obtained in
biomedicine in recent decades, it seems unusual that it took so long
before scientists and cardiologists began to systematically deal
with the impact of 2 kg of microorganisms that colonize us and live
with us "for better or worse". Although only some of the mechanisms
that link the gut microbiota and certain cardiovascular diseases are
presented, we must be aware of the possibilities of this research
area in the development of potential drugs in the future. The newly
clarified connections between dysbiosis and the pathogenesis of
cardiovascular diseases offer new possibilities for early and
targeted action.
P.S.Perhaps the new research will lead to a new subspecialization in
internal medicine, which, as prof. Miodrag
Krstićanecdotallymentionedin his lecture at the Congress of Internal
Medicine in 2019, will be calledgastroenterocardiology.
LITERATURE:
- Mengchao Jin Mengchao Jin, Zhiyuan Qian, Jiayu Yin, Weiting
Xu, and Xiang Zhou The role of intestinal microbiota in
cardiovascular disease Journal of Cellular and Molecular
Medicine 2019; 23(4): 2343–2350.
- Sekirov I, Russell SL, Antunes LC, Finlay BB. Gut microbiota
in health and disease. Physiol Rev. 2010;90(3):859-904. DOI:
10.1152/physrev.00045.2009 PMID: 20664075
- Tang WH, Kitai T, Hazen SL. Gut Microbiota in Cardiovascular
Health and Disease. Circ Res. 2017;120(7):1183-96. DOI:
10.1161/CIRCRESAHA.117.309715 PMID: 28360349
- David LA, Maurice CF, Carmody RN, Gootenberg DB, Button JE,
Wolfe BE, et al. Diet rapidly and reproducibly alters the human
gut microbiome. Nature. 2014;505(7484):559-63. DOI:
10.1146/annurev-med-060513-093205 PMID: 25587655
- Tamburini S, Shen N, Wu HC, Clemente JC. The microbiome in
early life: implications for health outcomes. Nat Med.
2016;22(7):713-22. DOI: 10.1038/nm.4142 PMID: 27387886
- Nallu A, Sharma S, Ramezani A, Muralidharan J, Raj D. Gut
microbiome in chronic kidney disease: challenges and
opportunities. Transl Res. 2017;179:24-37. DOI:
10.1016/j.trsl.2016.04.007 PMID: 27187743
- Tang WH, Wang Z, Levison BS, Koeth RA, Britt EB, Fu X, et
al. Intestinal microbial metabolism of phosphatidylcholine and
cardiovascular risk. N Engl J Med. 2013;368(17):1575-84. DOI:
10.1056/NEJMoa1109400 PMID: 23614584
- Ahmadmehrabi S, Tang WH. Gut microbiome and its role in
cardiovascular diseases. Curr Opin Cardiol. 2017;32(6):761-6.
DOI: 10.1097/HCO.0000000000000445 PMID: 29023288
- Yamashiro K, Tanaka R, Urabe T, Ueno Y, Yamashiro Y, Nomoto
K, et al. Gut dysbiosis is associated with metabolism and
systemic inflammation in patients with ischemic stroke. PLoS
One. 2017;12(2):e0171521. DOI: 10.1371/journal.pone.0171521 PMID:
28166278
- Vinod N. The Novel Dimensions of Cardio-Metabolic Health:
Gut Microbiota, Dysbiosis and its Fallouts. Curre Res Diabetes &
Obes J. 2019;11(1):555805. DOI: 10.19080/CRDOJ.2019.11.555805
- ANTAL, I., JELIĆ, M., SILA, S., KOLAČEK, S. i TAMBIĆ
ANDRAŠEVIĆ, A. LJUDSKA MIKROBIOTA I MIKROBIOM. Acta medica
Croatica, 2019;73(1),3-11. Preuzeto sa
https://hrcak.srce.hr/
- Lloyd-Price J, Abu-Ali G, Huttenhower C. The healthy human
microbiome. Genome Med 2016; 8 (1):51.
- Qi Y, Aranda JM, Rodriguez V, Raizada MK, Pepine CJ. Impact
of antibiotics on arterial blood pressure in a patient with
resistant hypertension - A case report. Int J Cardiol.
2015;201:157-8. DOI: 10.1016/j.ijcard.2015.07.078 PMID: 26301638
- Gomez-Arango LF, Barrett HL, McIntyre HD, Callaway LK,
Morrison M, Dekker Nitert M; SPRING Trial Group. Increased
Systolic and Diastolic Blood Pressure Is Associated With Altered
Gut Microbiota Composition and Butyrate Production in Early
Pregnancy. Hypertension. 2016;68(4):974-81. DOI:
10.1161/HYPERTENSIONAHA.116.07910 PMID: 27528065
- Niebauer J, Volk HD, Kemp M, Dominguez M, Schumann RR,
Rauchhaus M, et al. Endotoxin and immune activation in chronic
heart failure: a prospective cohort study. Lancet.
1999;353(9167):1838-42. DOI: 10.1016/S0140-6736(98)09286-1 PMID:
10359409
- Cani PD, Amar J, Iglesias MA, Poggi M, Knauf C, Bastelica D,
et al. Metabolic Endotoxemia Initiates Obesity and Insulin
Resistance. Diabetes. 2007;56(7):1761-72. DOI: 10.2337/db06-1491
PMID: 17456850
- Karlsson FH, Fåk F, Nookaew I, Tremaroli V, Fagerberg B,
Petranovic D, et al. Symptomatic atherosclerosis is associated
with an altered gut metagenome. Nat Commun. 2012;3(1):1245. DOI:
10.1038/ncomms2266 PMID: 23212374
- Wang Z. Roberts A.B. Buffa J.A. Levison B.S. Zhu W. Org E.
et al.Non-lethal inhibition of gut microbial trimethylamine
production for the treatment of therosclerosis.Cell.
2015;163:1585-1595.
- Townsend MK, Aschard H, De Vivo I, Michels KB, Kraft P
Genomics, telomere length, epigenetics, and metabolomics in the
nurses’ health studies. Am J Public Health 2016;106(9):
1663-1668.
- Ju Seung Kwun, Si-Hyuck Kang, Hyo-Jung Lee, Chang-Hwan Yoon,
Jung-Won Suh, Young-Seok Cho, METAGENOMIC ANALYSIS OF MICROBIOTA
IN PATIENTS WITH ST-SEGMENT ELEVATION MYOCARDIAL INFARCTION 2.
ORAL, GUT, AND THROMBUS MICROBIOME IN ST-SEGMENT ELEVATION
MYOCARDIAL INFARCTION 112JACC, 2020;75(11). Preuzeto 18.11.2020
sa
https://www.jacc.org/doi/pdf/10.1016/S0735-1097%2820%2930739-7
- Yamashiro K, Tanaka R, Urabe T, Ueno Y, Yamashiro Y, et al.
Gut dysbiosis is associated with metabolism and systemic
inflammation in patients with ischemic stroke. PLoS One
2017;12(2): e0171521.
- Honour J The possible involvement of intestinal bacteria in
steroidal hypertension. Endocrinology 1982;110(1): 285-287.
- Yang T, Santisteban MM, Rodriguez V, Li E, Ahmari N, et al.
Gut dysbiosis is linked to hypertension. Hypertension
2015;65(6):1331-1340.
- Karbach SH, Schonfelder T, Brandao I, Wilms E, Hörmann N, et
al. Gut microbiota promotes angiotensin II-induced arterial hy
pertension and vascular dysfunction. J Am Heart Assoc 2016;5(9):
e003698.
- Koh A, De Vadder F, Kovatcheva-Datchary P, Backhed F From
dietary fiber to host physiology: short-chain fatty acids as key
bacterial metabolites. Cell 2016;165(6): 1332-1345.
- Rey FE, Faith JJ, Bain J, Muehlbauer MJ, Stevens RD, et al.
Dissecting the in vivo metabolic potential of two human gut
acetogens. J Biol Chem 2010;285(29): 22082-22090.
- Louis P, Flint HJ Formation of propionate and butyrate by
the human colonic microbiota. Environ Microbiol 2017;19(1):
29-41.
- Duncan SH, Barcenilla A, Stewart CS, Pryde SE, Flint HJ
Acetate utilization and butyryl coenzyme A (CoA): acetate-CoA
transferase in butyrate-producing bacteria from the human large
intestine. Appl En- viron Microbiol 2002;68(10): 5186-5190.
- Gomez-Arango LF, Barrett HL, McIntyre HD, Callaway LK,
Morrison M, et al. Increased systolic and diastolic blood
pressure is associ- ated with altered gut microbiota composition
and butyrate production in early pregnancy. Hypertension
2016;68(4): 974-981.
- Marques FZ, Nelson E, Chu PY, Horlock D, Fiedler A, et al.
High-fiber diet and acetate supplementation change the gut
microbi- ota and prevent the development of hypertension and
heart failure in hypertensive mice. Circulation 2017;135(10):
964-977.
- Tan JK, McKenzie C, Marino E, Macia L, Mackay CR
Metabolite-sensing g protein-coupled receptors-facilitators of
diet-related immune regulation. Annu Rev Immunol 2017;35:
371-402.
- Pluznick JL, Protzko RJ, Gevorgyan H, Peterlin Z, Sipos A,
et al. Olfactory receptor responding to gut microbiota-derived
signals plays a role in renin secretion and blood pressure
regulation. Proc Natl Acad Sci U.S.A 2013;110(11): 4410-4415.
- Natarajan N, Hori D, Flavahan S, Steppan J, Flavahan NA, et
al. Microbial short chain fatty acid metabolites lower blood
pressure via endothelial G protein-coupled receptor 41. Physiol
Genomics 2016;48(11): 826-834.
- Miyamoto J, Kasubuchi M, Nakajima A, Irie J, Itoh H, et al.
The role of short-chain fatty acid on blood pressure regulation.
Curr Opin Nephrol Hypertens 2016;25(5): 379-383.
- Packer CS, Rice AE, Johnson TC Oxidized low density
lipoprotein (OX-LDL) induced arterial muscle contraction
signaling mechanisms. Open Hyperten J 2014;6: 20-26.
- Ma J, Li H. The Role of Gut Microbiota in Atherosclerosis
and Hypertension. Front Pharmacol. 2018;9:1082. DOI:
10.3389/fphar.2018.01082 PMID: 30319417
- Ott SJ, El Mokhtari NE, Musfeldt M, Hellmig S, Freitag S,
Rehman A, et al. Detection of diverse bacterial signatures in
atherosclerotic lesions of patients with coronary heart disease.
Circulation. 2006;113(7):929-37. DOI:
10.1161/CIRCULATIONAHA.105.579979 PMID: 16490835
- Lam V, Su J, Koprowski S, Hsu A, Tweddell JS, Rafiee P, et
al. Intestinal microbiota determine severity of myocardial
infarction in rats. FASEB J. 2012;26(4):1727-35. DOI:
10.1096/fj.11-197921 PMID: 22247331
- Lam V, Su J, Hsu A, Gross GJ, Salzman NH, Baker JE.
Intestinal Microbial Metabolites Are Linked to Severity of
Myocardial Infarction in Rats. PLoS One. 2016;11(8):e0160840.
DOI: 10.1371/journal.pone.0160840 PMID: 27505423
- Gan XT, Ettinger G, Huang CX, Burton JP, Haist JV,
Rajapurohitam V, et al. Probiotic administration attenuates
myocardial hypertrophy and heart failure after myocardial
infarction in the rat. Circ Heart Fail. 2014;7(3):491-9. DOI:
10.1161/CIRCHEARTFAILURE.113.000978 PMID: 24625365
- Patterson E., Cryan J. F., Fitzgerald G. F., Ross R. P.,
Dinan T. G., Stanton C. Gut microbiota, the pharmabiotics they
produce and host health. Proceedings of the Nutrition Society.
2014;73(4):477–489. doi: 10.1017/S0029665114001426.
- Pasini E., Aquilani R., Testa C., et al. Pathogenic gut
flora in patients with chronic heart failure. JACC: Heart
Failure. 2016;4(3):220–227. doi: 10.1016/j.jchf.2015.10.009
- Luedde M., Winkler T., Heinsen F. A., et al. Heart failure
is associated with depletion of core intestinal microbiota. ESC
Heart Failure. 2017;4(3):282–290. doi: 10.1002/ehf2.12155.
- Kamo T., Akazawa H., Suda W., et al. Dysbiosis and
compositional alterations with aging in the gut microbiota of
patients with heart failure. PLoS One. 2017;12(3):e0174099. doi:
10.1371/journal.pone.0174099.
- Kummen M., Mayerhofer C. C. K., Vestad B., et al. Gut
microbiota signature in heart failure defined from profiling of
2 independent cohorts. Journal of the American College of
Cardiology. 2018;71(10):1184–1186. doi:
10.1016/j.jacc.2017.12.057.
- Arpaia N., Campbell C., Fan X., et al. Metabolites produced
by commensal bacteria promote peripheral regulatory T-cell
generation. Nature. 2013;504(7480):451–455. doi:
10.1038/nature12726
- Kitai T, Kirsop J, Tang WH. Exploring the Microbiome in
Heart Failure. Curr Heart Fail Rep. 2016;13(2):103-9. DOI:
10.1007/s11897-016-0285-9 PMID: 26886380
- Tang WHW, Wang Z, Fan Y, Levison B, Hazen JE, Donahue LM, et
al. Prognostic Value of Elevated Levels of Intestinal
Microbe-Generated Metabolite trimethylamine-N-oxide in Patients
With Heart Failure: Refining the Gut Hypothesis. J Am Coll
Cardiol. 2014;64(18):1908-14. DOI: 10.1016/j.jacc.2014.02.617
PMID: 25444145
- Skok P, Skok K. Prebavna cev in srčno-žilne bolezni – ali
imajo kaj skupnega? Zdrav Vestn. 2020;89(9–10):528–38.
- Bäckhed F, Ding H, Wang T, Hooper LV, Koh GY, et al. The gut
microbiota as an environmental factor that regulates fat
storage. Proc Natl Acad Sci USA 2004;101(4):15718-15723.
- Turnbaugh PJ, Ley RE, Mahowald MA, Magrini V, Mardis ER, et
al. An obesity-associated gut microbiome with increased capacity
for energy harvest. Nature 2006;444(7122): 1027-1031.
- Samuel BS, Shaito A, Motoike T, Rey FE, Backhed F, et al.
Effects of the gut microbiota on host adiposity are modulated by
the short- chain fatty-acid binding G protein-coupled receptor,
Gpr41. Proc Natl Acad Sci USA 2008;105(43): 16767-16772.
- Bäckhed F, Manchester JK, Semenkovich CF, Gordon JI Mecha
nisms underlying the resistance to diet-induced obesity in
germ-free mice. Proc Natl Acad Sci USA 2007;104(3): 979-984.
- Ley RE, Bäckhed F, Turnbaugh P, Lozupone CA, Knight RD, et
al. Obesity alters gut microbial ecology. Proc Natl Acad Sci USA
2005;102: 11070-11075.
- Cani PD, Amar J, Iglesias MA, Poggi M, Knauf C, et al.
Metabolic endotoxemia initiates obesity and insulin resistance.
Diabetes 2007;56(7):1761-1772.
- de La Serre CB, Ellis CL, Lee J, Hartman AL, Rutledge JC, et
al. Propensity to high-fat diet-induced obesity in rats is
associated with changes in the gut microbiota and gut
inflammation. Am J Physiol Gas- trointest Liver Physiol
2010;299(4): G440-G448.
- Harris K, Kassis A, Major G, Chou CJ Is the gut microbiota a
new factor contributing to obesity and its metabolic disorders?.
J Obes 2012;2012: 879151. doi: 10.1155/2012/879151.
- Jia Q, Li H, Zhou H, Zhang X, Zhang A, Xie Y, et al.
Microbiota and irritable bowel syndrome: A critical inventory.
Cardiovasc Ther. 2019;2019:5164298. DOI: 10.1155/2019/5164298
PMID: 31819762
- Salehi-Abargouei A, Maghsoudi Z, Shirani F, Azadbakht L.
Effects of Dietary Approaches to Stop Hypertension (DASH)-style
diet on fatal or nonfatal cardiovascular diseases—incidence: a
systematic review and meta-analysis on observational prospective
studies. Nutrition. 2013;29(4):611-8. DOI:
10.1016/j.nut.2012.12.018 PMID: 23466047
- Rifai L, Pisano C, Hayden J, Sulo S, Silver MA. Impact of
the DASH diet on endothelial function, exercise capacity, and
quality of life in patients with heart failure. Proc Bayl Univ
Med Cent. 2015;28(2):151-6. DOI: 10.1080/08998280.2015.11929216
PMID: 25829641
- Lopez-Garcia E, Rodriguez-Artalejo F, Li TY, Fung TT, Li S,
Willett WC, et al. The Mediterranean-style dietary pattern and
mortality among men and women with cardiovascular disease. Am J
Clin Nutr. 2014;99(1):172-80. DOI: 10.3945/ajcn.113.068106 PMID:
24172306
- De Filippis F, Pellegrini N, Vannini L, Jeffery IB, La
Storia A, Laghi L, et al. High-level adherence to a
Mediterranean diet beneficially impacts the gut microbiota and
associated metabolome. Gut. 2016;65(11):1812-21. DOI:
10.1136/gutjnl-2015-309957 PMID: 26416813
- M. Trøseid et alThe gut microbiome in coronary artery
disease and heart failure: Current knowledge and future
directions Review| 2020;52:102649. Published: February 18, 2020
DOI:https://doi.org/10.1016/j.ebiom.2020.102649
- Hill C. Guarner F. Reid G. Gibson G.R.Merenstein D.J. Pot B.
et al. Expert consensus document. the international scientific
association for probiotics and prebiotics consensus statement on
the scope and appropriate use of the term probiotic.Nat Rev
Gastroenterol Hepatol. 2014;11:506-514.
- Patel R. DuPont H.L. New approaches for bacteriotherapy:
prebiotics, new-generation probiotics, and synbiotics. Clin
Infect Dis. 2015; 60: S108-S121.
- Lam V. Su J. Koprowski S. Hsu A. Tweddell J.S. Rafiee P. et
al. Intestinal microbiota determine severity of myocardial
infarction in rats. FASEB J. 2012; 26: 1727-1735.
- Gan X.T. Ettinger G. Huang C.X. Burton J.P. Haist J.V.
Rajapurohitam V. et al. Probiotic administration attenuates
myocardial hypertrophy and heart failure after myocardial
infarction in the rat. Circ Heart Fail. 2014; 7: 491-499.
- Costanza A.C. Moscavitch S.D. Faria Neto H.C. Mesquita E.T.
Probiotic therapy with saccharomyces boulardii for heart failure
patients: a randomized, double-blind, placebo-controlled pilot
trial. Int J Cardiol. 2015; 179: 348-350.
- He Z. Wang J. Chen Y. Cong X. Li N. Ding R. et al. Potential
risk associated with direct modulation of the gut flora in
patients with heart failure. ESC Heart Fail. 2019; 6: 555-556.
- Mayerhofer C.C.K. Awoyemi A. Hov J.R. Troseid M. Broch K.
Reply: potential risk associated with direct modulation of the
gut flora in patients with heart failure.ESC Heart Fail. 2019;
6: 557-558.
- Yelin I. Flett K.B. Merakou C. Mehrotra P. Stam J. Snesrud
E. et al. Genomic and epidemiological evidence of bacterial
transmission from probiotic capsule to blood in ICU patients.
Nat Med. 2019; 25: 1728-1732.
- Gibson G.R. Hutkins R. Sanders M.E. Prescott S.L. Reimer
R.A. Salminen S.J. et al. Expert consensus document: the
international scientific association for probiotics and
prebiotics (ISAPP) consensus statement on the definition and
scope of prebiotics. Nat Rev Gastroenterol Hepatol.
2017;14:491-502.
- Chambers E.S. Preston T. Frost G. Morrison D.J. Role of gut
microbiota-generated short-chain fatty acids in metabolic and
cardiovascular health.Curr Nutr Rep. 2018; 7: 198-206.
- Johnson L.P. Walton G.E. Psichas A. Frost G.S. Gibson G.R.
Barraclough T.G. Prebiotics modulate the effects of antibiotics
on gut microbial diversity and functioning in vitro. Nutrients.
2015; 7: 4480-4497.
- Chambers E.S. Byrne C.S. Morrison D.J. Murphy K.G. Preston
T. Tedford C. et al. Dietary supplementation with inulin-propionate
ester or inulin improves insulin sensitivity in adults with
overweight and obesity with distinct effects on the gut
microbiota, plasma metabolome and systemic inflammatory
responses: a randomised cross-over trial. Gut. 2019; 68:
1430-1438.
- Lin PP, Hsieh YM, Kuo WW, Lin YM, Yeh YL, Lin CC, et al.
Probiotic-fermented purple sweet potato yogurt activates
compensatory IGFIR/PI3K/Akt survival pathways and attenuates
cardiac apoptosis in the hearts of spontaneously hypertensive
rats. Int J Mol Med. 2013;32(6):1319-28. DOI:
10.3892/ijmm.2013.1524 PMID: 24127171
- Tiihonen K, Tynkkynen S, Ouwehand A, Ahlroos T, Rautonen N.
The effect of ageing with and without non-steroidal
anti-inflammatory drugs on gastrointestinal microbiology and
immunology. Br J Nutr. 2008;100(1):130-7. DOI:
10.1017/S000711450888871X PMID: 18279548
- Zhou X, Li J, Guo J, Geng B, Ji W, Zhao Q, et al.
Gut-dependent microbial translocation induces inflammation and
cardiovascular events after ST-elevation myocardial infarction.
Microbiome. 2018;6(1):66. DOI: 10.1186/s40168-018-0441-4 PMID:
29615110
- Conraads V.M. Jorens P.G.De Clerck L.S.van Saene H.K. Ieven
M.M. et al. Selective intestinal decontamination in advanced
chronic heart failure: a pilot trial.Eur J Heart Fail. 2004; 6:
483-491.
- Fox M.APeterson S. Fabri B.M. van Saene H.K. Selective
decontamination of the digestive tract in cardiac surgical
patients. Crit Care Med. 1991; 19: 1486-1490.
- Tang T.W.H. Chen H.C. Chen C.Y. Yen C.Y.T. Lin C.J.
Prajnamitra R.P. et al. Loss of gut microbiota alters immune
system composition and cripples postinfarction cardiac repair.
Circulation. 2019; 139: 647-659.
- Lam V. Su J. Koprowski S. Hsu A. Tweddell J.S. Rafiee P. et
al.Intestinal microbiota determine severity of myocardial
infarction in rats.FASEB J. 2012; 26: 1727-1735.
- Lam V. Su J. Hsu A. Gross G.J. Salzman N.H. Baker J.E.
Intestinal microbial metabolites are linked to severity of
myocardial infarction in rats. PLoS ONE. 2016; 11:e0160840.
- Andraws R. Berger J.S. Brown D.L. Effects of antibiotic
therapy on outcomes of patients with coronary artery disease: a
meta-analysis of randomized controlled trials.JAMA. 2005; 293:
2641-2647.
- Winkel P. Hilden J. Hansen J.F. Kastrup J. Kolmos H.J.
Kjoller E.et al. Clarithromycin for stable coronary heart
disease increases all-cause and cardiovascular mortality and
cerebrovascular morbidity over 10years in the claricor
randomised, blinded clinical trial. Int J Cardiol. 2015; 182:
459-465.
- Knoop K.A.McDonald K.G. Kulkarni D.H. Newberry R.D.
Antibiotics promote inflammation through the translocation of
native commensal colonic bacteria. Gut. 2016; 65: 1100-1109.
- Colman RJ, Rubin DT Fecal microbiota transplantation as
therapy for inflammatory bowel disease: a systematic review and
meta-analysis. J Crohns Colitis 2014;8(12): 1569-1581.
- Vrieze A, Van Nood E, Holleman F, Salojärvi J, Kootte RS, et
al. Transfer of intestinal microbiota from lean donors increases
insulin sensitivity in individuals with metabolic syndrome.
Gastroenterolo- gy 2012;143(4): 913-916.
- Petrof E, Gloor G, Vanner S, Weese SJ, Carter D, et al.
Stool substitute transplant therapy for the eradication of
Clostridium difficile infection: ‘repopulating’ the gut.
Microbiome 2013;1(1): 3.
|
|
|
|